Abstract
The nanoparticle Au/TiO2 embedded system plays a very important role in the plasmonic solar cell. The features of the nanoparticle embedded system will determine light enhancement, light absorption, scattering and localized surface plasmon resonance (LSPR), aiming to enhance the efficiency of the plasmatic solar cell. The characterizations of nanoparticles Au/TiO2 embedded system consist of many parameters: the sizes of nanoparticles (Au, TiO2), the weight ratio of Au to TiO2, the thickness of the single layer or multilayer of Au/TiO2, the arrangements of Au and TiO2 nanoparticles in integrated-matrix system, the light absorption, scattering and LSPR capacities of the Au/TiO2 system. These parameters, however, depend on the technological conditions, the structure of plasmonic solar cell as well as the used substrate materials. This paper presents some technological developments for nanoparticles Au/TiO2 embedded systems by different methods, including the preparation of the mixer Au/TiO2 solutions and fabrication of the nanoparticle Au/TiO2 systems with different Au percentages on several substrates (glass/ITO and AAO(Al)/Si...), and measured results of the morphological, structural and optical properties using FESEM, EDX, UV–vis spectroscopy. The comparisons of experiment results between different technology conditions and substrates (glass/ITO, AAO(Al)/Si...) are also shown and discussed with the aim of choosing the suitable technological process and technological conditions for application in the plasmonic solar cell.
Export citation and abstract BibTeX RIS

Original content from this work may be used under the terms of the Creative Commons Attribution 3.0 licence. Any further distribution of this work must maintain attribution to the author(s) and the title of the work, journal citation and DOI.
1. Introduction
Currently, one of the serious challenges to mankind is to ensure energy security or energy sustainability. This challenge has to be answered with a low-cost solution to produce renewable energy sources, where process-technology harvesting of the power of the Sun with photovoltaic technologies appears to be the only reasonable large-scale answer to the energy challenges [1–5]. To date there have been three developed solar cells generations [3, 5–7], among them the plasmonic structural solar cell is a type belonging to the third generation, which is a promising new type. The most important structure in the plasmonic solar cells is the integrated noble metal nanoparticle (Au (Ag)/ TiO2 (ZnO...) system [3–5, 7]. This structure, the so-called plasmonic nanostructure, could support the formation of the surface plasmon resonance in response to a photon flux, localizing electromagnetic energy close to the surface. So far, plasmonic solar cells that have been recently developed are at the beginning stage and have many research issues, including both theoretical and practical problems that must be overcome [4, 5, 8–10]. In our previous works we have reported about plasmonics solar cell, and the difficulties and challenges for development in the near future. We have also designed several structures of plasmonic solar cells and experimentally prepared some samples of plasmonic solar cells, investigated the structural, electrical and optical properties; however, the obtained results of electrical and optical properties are still very limited [10, 11]. In the present work, we prepare nanoparticles Au/TiO2 embedded systems by different technological methods on different substrates, study the structural properties of the prepared Au/TiO2 layers using scanning electron microscopy (SEM), transmission electron spectroscopy (TEM), atomic force microscopy (AFM), energy dispersive x-ray spectroscopy (EDX) techniques etc, and investigate the localized surface plasmon resonance (LSPR) by measuring absorption coefficients. Based on the results of the comparison of their characterizations we could choose the suitable technological methods for application in plasmonic solar cell preparation.
2. Experimental
2.1. The substrates, Au, TiO2 solutions and equipment used in experiments
In the experiments we have used the standard 1 × 2 cm2 Lama glass and Sigma Aldrid glass/ITO substrates. ITO layer on glass has a thickness of about 600 nm; its sheet resistance is 30–40 Ω/square. We use also anodic aluminum oxide (AAO) template on Si substrates. We use pure TiO2 powder with sizes in the range of 20–50 nm and Au nanoparticle size in the range of 10–20 nm (figure 1(a)). We prepared Au/TiO2 solution with different Au percentages of 5%, 10% from the TiO2 solution (30 g liter−1) and nanoparticles 1 mM Au water environment sol, which has a concentration of 0.2 g liter−1, and pH is about 5. In order to get a desired density of Au/TiO2 sol (5%, 10%...), we have to mix different 5:95, 10:90... weight ratios of nanoparticle Au sol and TiO2 sol, then we perform heat treatment at 50–70 °C low temperature to get higher densities solutions. The detailed technological processes can be seen in [10, 11]. The spin-on equipment used in our experiments has controllable speed from 0 rpm to 5000 rpm. We use an S-4800 scanning microscope, D-5000 x-ray diffractometer, α-step and varian cary 5000 UV/Vis/NIR spectrophotometer for measurements of FE-SEM, EDX, thin film thickness and absorption coefficiency, respectively.
Figure 1. Optical image of the different solutions in small bottles: nanoparticlesTiO2 solutions with sizes in range of from 20–50 nm and the prepared different Au(5%)/TiO2, Au(10%)/TiO2 solutions with Au sizes in range of from 10–20 nm for producing nanoparticles Au/TiO2 embedded layers on different substrates (a); the measured absorption coefficiencies of Au(5%)/TiO2 and Au(10%)/TiO2 liquid solution (b).
Download figure:
Standard image High-resolution imageIn order to ensure using the different Au percentage solutions we have investigated the absorption coefficiencies of Au(5%)/TiO2 and of Au(10%)/TiO2 liquid solutions being in tubes. The obtained results are shown in figure 1(b). Here we can see that the absorption coefficiencies dominate with considerably high peaks at wave length region around 500 nm characterizing Au spheres/TiO2.
2.2. The main technological processes for producing nanoparticles Au/TiO2 embedded systems and the investigation of morphologies, absorption of the glass/Au/TiO2 experiment samples
We have carried out the technological processes for preparation of nanoparticle Au/TiO2 embedded systems by different technologies: spin coating TiO2 on glass/ITO substrate; spin coating Au(5%)/TiO2 and Au(10%)/TiO2 on glass and glass/ITO substrates; performing the evaporation Au-heat treatment then spin coating TiO2 on glass/ITO substrate, dropping-spreading Au/TiO2 on glass/ITO substrate and spin coating Au/TiO2 onto AAO template on Si substrate. The detailed technology process was reported in [10, 11]. The main technology steps for producing Au/TiO2 embedded layers on the different substrates can be summarized briefly as follows (figure 2):
- -Choose suitable substrates (glass/ITO, N+N Si, AAO/Si... substrates),
- -Carry out the chemical treatments for substrates using standard technology,
- -Perform Au evaporation or sputtering to the substrates with thickness in the range of 20–30 nm and then thermally treat at 300–350 °C in air environment for 60–90 mins,
- -Perform spin coating the TiO2 solution or Au/TiO2 solutions with different Au percentages on the substrates. A nanoparticle Au/TiO2 layer must have thickness in the range of 100–500 nm. Au nanoparticles and TiO2 nanoparticles sizes must be in the range of 10–20 nm and 20–50 nm, respectively. After spin coating, thermal treatment was carried out at 300–500 °C in air for 30–60 mins depending on the technological process: Au evaporation layer-TiO2 spin coating technology or the mix Au/TiO2 sol technology on substrates.
- -Investigate morphology of Au/TiO2 embedded layer using SEM and AFM measurements,
- -Investigate absorption coefficiencies of Au/TiO2 embedded layers using varian cary 5000 UV/Vis/NIR spectrophotometer.
Figure 2. The layers structure of sample used in our experiments (a), and the designed layers structure of plasmonic solar cell for fabrication (b).
Download figure:
Standard image High-resolution image3. The experimental results of nanoparticle TiO2 layer, nanoparticles Au/TiO2 embedded systems and their corresponding absorption coefficiencies
The results demonstrated in figures 3 to 12 show morphologies of nanoparticles TiO2 layers, nanoparticles Au/TiO2 embedded systems prepared by different technologies on different substrates, including their corresponding absorption coefficiencies of samples containing Au/TiO2 layers. Each figure caption contains the technological method and technological conditions.
Figure 3. SEM images of the 20–30 nm size TiO2 (PSA-01) by spin coating onto ITO/glass with speed 500 rpm for 15 s then by continuous spin with speed 2000 rpm for 30 s, heat treatment at 500 °C in air environment for 30 min (a), (b); x-ray diffraction pattern shows TiO2 layer has rutine structure before heat treatment (R) and has anatase (A) structure after heat treatment (c), AFM image (d) [10, 11].
Download figure:
Standard image High-resolution image4. Discussions
We know that the light scattering of small nanoparticles is of special research interest today for a variety of applications. For the energy sector where they are used to increase the efficiency of solar cells, the way these nanoparticles scatter light is of vital importance to the research [3, 5, 7, 11–17]. Light scattering is, in general, the attenuation of a beam of light by particles, either by absorption or scattering. The sum of these two parts is known as the extinction. For nanoparticles with diameters well below the wavelength of light, a point dipole model well describes the absorption and scattering of light. The scattering and absorption cross-sections, Cscat and Cabs, respectively, are given by following formulae [14]:
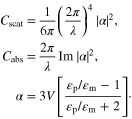
Here V is the particle volume, p is the dielectric function of the particle and
m is the dielectric function of the embedding medium. We can see that when
the particle polarizability will become very large. This is known as the surface plasmon resonance. This is sometimes called the dipole plasmon resonance of the particle. At the surface plasmon resonance the scattering cross-section can well exceed the geometrical cross section of the particle, consequently the absorption coefficienct versus wavelength will be enhanced in a certain range of wavelength depending on nanoparticles system being on the substrate. The experiment results showed that the enhanced absorption coefficient of Au/TiO2 spherical nanoparticles has dominated strongly in the range of 500–600 nm [3, 5–7, 12–14, 16, 17].
Concerning our obtained results, in general we can see that the Au/TiO2 layers contain separate clusters on the substrates as shown in figures 4–6. The enhancement of absorption coefficiencies are not shown in those samples; meanwhile, the results demonstrated in figures 9–12 where Au/TiO2 layers with large thickness, high density and uniformity without clusters have shown enhancements of absorption coefficiencies in the wavelength range 500–700 nm. In these cases the LSPR has demonstrated its effect. It is also worth noting that the absorption coefficiencies of Au(5%)/TiO2 and of Au(10%)/TiO2 liquid solutions (figure 1(b)) are also strongly enhanced in the wavelength range 500–600 nm (this can be also seen in figure 9(c)); meanwhile the absorption coefficiencies of all other samples containing Au/TiO2 layers in figures 10(c), 11(b) and (d), and 12(c) are shifted and enhanced in the wavelength range of 600–700 nm. These facts show that LSPR effect takes place in these samples.
Figure 4. SEM images of Au (5%)/TiO2 by spin coating onto ITO/glass with speed 500 rpm for 15 s then by continuous spin with speed 2000 rpm for 30 s, not yet heat treatment. Au (5%)/TiO2 is still wet-liquid sol (a), Au/TiO2 layer are separately clustered (b).
Download figure:
Standard image High-resolution imageFigure 5. SEM images of Au(5%)/TiO2 obtained by spin coating onto ITO/glass with speed 500 rpm for 15 s then by continuous spin with speed 2000 rpm for 30 s, after that heat treatment carried out at 500 °C in air environment for 30 min. Au (5%)/TiO2 are more separated (a), Au/TiO2 clusters are magnified (b).
Download figure:
Standard image High-resolution imageFigure 6. SEM images of Au(10%)/TiO2 obtained by spin coating onto ITO/glass with speed 500 rpm for 15 s then by continuous spin with speed 2000 rpm for 30 s, after that heat treatment at 500 °C in air environment for 30 min. Au (10%)/TiO2 are still separated (a), Au/TiO2 clusters are magnified (b).
Download figure:
Standard image High-resolution imageFigure 7. The typical cross-section SEM images on Au/TiO2 clusters by spin coating onto ITO/glass with speed 500 rpm for 15 s then by continuous spin with speed 2000 rpm for 30 s, after that heat treatment at 500 °C in air environment for 30 min. The thickness of Au/TiO2 is about 150 nm (top layer), ITO layer thickness is about 600 nm (middle) and glass layer (bottom). SEM cross-section of Au (5%)/TiO2 cluster region (a), and SEM cross-section of Au (10%)/TiO2 cluster region (b).
Download figure:
Standard image High-resolution imageFigure 8. The original absorption curves measured on seven samples with different Au percentages and different spin coating speeds on glass/ITO substares, among them there are three absorption coefficiencies of three samples: black curve for Au (0%) TiO2 (on figure 3), red curve for Au (5%)/TiO2 (figure 5) and blue curve for Au (10%)/TiO2 (figure 6). The results showed that there are not enhanced absorptions in the region around 500 nm wavelength, also meaning that the localized surface plasmon resonance has not yet dominated in these samples. The separated clusters of Au/TiO2 on substrates are the main reason for lack of absorption enhancement.
Download figure:
Standard image High-resolution imageFigure 9. Optical image (a) and SEM image (b) of nanoparticles Au (5%)/TiO2 layer by spin coating onto the glass/TiO2 substrate, heat treated at 500 °C for 30 min and result of measured absorption spectrum of the Au (5%)/TiO2 layer. The absorption spectrum is enhanced in the range of 500–600 nm; this shows the effect of LSPR.
Download figure:
Standard image High-resolution imageFigure 10. SEM images of Au nanoparticles evaporated onto glass/TiO2 substrate then heat treated at 350 °C in air environment for 60 mins (a), SEM image of the nano Au (5%)/TiO2 solution spin coated onto the Au evaporated glass/TiO2 substrate heat treated at 500 °C for 30 min (b) and the results of measured absorption spectrum of the Au (5%)/TiO2 layer. The absorption spectrum is enhanced in the range of 500 nm and 700 nm; this shows the effect of LSPR.
Download figure:
Standard image High-resolution imageFigure 11. SEM image of a nanoparticle Au (5%)/TiO2 layer by dropping-spreading Au/TiO2 on glass/ITO substrate onto the glass/TiO2 substrate, heat treated at 500 °C for 30 min (a), and result of measured absorption spectrum of this sample (b). SEM image of the nanoparticle Au (10%)/TiO2 layer by dropping-spreading Au/TiO2 on glass/ITO substrate onto the glass/TiO2 substrate, heat treated at 500 °C for 30 mins (c) and result of measured absorption spectrum of this sample (d). Both the absorption coefficiencies of two samples show the enhancement in the range of 600–700 nm; these show the effect of LSPR.
Download figure:
Standard image High-resolution imageFigure 12. SEM image of AAO(Al)/Si substrate with regular pores (a) and SEM image of the nanoparticle Au(5%)/TiO2 layer on AAO(Al)/Si substrate by spin coated with spin speed 1000 rpm for 50 s (b) and the result of measured absorption spectrum of this sample (c). The absorption spectrum shows the enhancement in the range of 600–700 nm; this means the effect of LSPR.
Download figure:
Standard image High-resolution image5. Conclusions
We have fabricated 20–50 nm nanoparticle TiO2 layers and nanoparticle Au/TiO2 embedded layers with different Au percentages on different substrates (glass, glass/ITO, AAO/Si) by different technological processes with different technological conditions: spin coating TiO2 on glass/ITO substrate; spin coating Au(5%)/TiO2 layers and Au(10%)/TiO2 layers onto glass and glass/ITO substrates; we have carried out the Au evaporation, heat treatment and then spin coating TiO2 on glass/ITO substrate. We have also carried out the process of dropping-spreading Au/TiO2 on glass/ITO substrate and spinning Au/TiO2 on AAO/Si substrates. On the basis of the obtained experimental results we could propose a good technological process of preparing good Au/TiO2 embedded system for applying in plasmonic solar cell preparation. In order to get higher absorption coefficiency or LSPR enhancement of nanoparticle Au/TiO2 embedded system we have to choose the suitable Au nanoparticle size to be around 10 nm and the size of TiO2 nanoparticles in the range 20–50 nm. We have to choose the suitable substrate material and also choose the optimal technological process: technology process consisting of Au evaporation onto substrate, heat treatment at 350 °C and then spin coating of Au/TiO2 with suitable speed to ensure high thickness of 2–3 μm, with a density of Au percentage about 10%, uniform Au/TiO2 layer without clusters on the surface.
Acknowledgments
The authors would like to express their gratitude to the NAFOSTED for financial funding of the basic research project with code 103.02-2013.14 in the period from 2013–2015, also many thanks for the support of Institute of Material Science, Vietnam Academy of Science and Technology.