Abstract
Due to increasing resistance of microorganisms towards current antibiotics, there is a need for new or enhanced antibiotics. Nanotechnology is a technology that enhances the use of gold nanoparticles (AuNP) in area of medical applications, especially as a drug carrier for targeted drug delivery. In this research, AuNPs was synthesized using biological method via bioreduction of Piper guineense aqueous leaf extract on tetra gold chloride, characterized using UV–Vis spectrophometer, DLS, TEM/EDS and FTIR. The synthesized AuNPs was covalently functionalized with polyethylene glycol and encapsulated with Lincomycin and in vitro dissolution methods was used to evaluate the potential performance of the formulated nanodrug. The nanodrug has highest release efficiency at the 9th minutes (23.4 mg ml−1 for 40 °C) and (29.5 mg ml−1 for 60 °C) compared with the non-nanodrug. The antibacterial potential of the nanodrug was seen on the gram-positive bacteria of Staphylococcus aureus and Streptococcus pyogenes with highest inhibitions of 18 mm (at 40 °C) and 16 mm (at 60 °C) for S. aureus, and 16 mm for S. pyogenes (both at 40 °C and 60 °C). The bacteria growth inhibition continued and lasted for 15 min, while that of non-nanodrug lasted for 9 min with lesser growth inhibition compared to the formulated nanodrug. This work shows that the presence of the AuNPs increased the release efficiency of lincomycin even at a lower concentration and also bacteria growth inhibition thereby suggesting the effectiveness of the nanodrug formulation.
Export citation and abstract BibTeX RIS

Original content from this work may be used under the terms of the Creative Commons Attribution 3.0 licence. Any further distribution of this work must maintain attribution to the author(s) and the title of the work, journal citation and DOI.
1. Introduction
The advances in functionalization chemistry with novel nano-materials and their diversified applications in the treatment of various human diseases have been in focus globally. Nanotechnology is fundamental for drug delivery, with many latent applications in clinical medicine and research [1]. The wide applications of nanotechnology in the field of biomedicine are enormous such as delivery of pharmaceuticals, diagnostic approaches as well as for therapeutic purposes [2]. The choice of nanoparticles for drug delivery is highly favoured by their unique chemical and physical properties that hold support for future development of treatment of diseases in drug delivery system with minimal side effect [3]. Nanoparticles are particles used in nanotechnology, with size range of 1–100 nm and have completely novel or advanced properties due to their high ratio of surface area to particle size as opposed to their larger counterparts [4]. Metallic nanoparticles are the subject of research efforts as new platforms for the target-specific delivery of therapeutic agents.
Nanocarriers are novel materials platform for target/specific delivery of therapeutic agents [5]. Through the years several delivery means were designed based on different nanomaterials, such as polymers [6], liposomes [7], dendrimers [8], nanorods [9], and nanotubes [10]. However, gold nanoparticles (AuNPs) have been identified as an attractive candidate for delivery into their targets [11]. The acceptance of AuNPs as an excellent candidate for drug delivery was due to its unique properties especially in the transport and release of the therapeutic agents to the target site [12]. The therapeutic agents to be delivered could be small drug molecules or large biomolecules, like proteins, DNA, or RNA and effectiveness of their release is a prerequisite for efficient therapy [13]. The release of therapeutic agents depend on different factors such as internal (glutathione (GSH) [14], or pH [15] or external (light) [16] stimuli. The internal stimuli control biological medium and the external stimuli provide spatial control over the release [13]. Li et al reported on using AuNPs as a carrier to antitumor, antibiotics and other antibacterial therapeutic agents [17]. Selvaraj and Alagar reported that a colloidal gold conjugate of the antileukemic drug 5-fluorouracil exhibited observable antibacterial and antifungal activities against S. aureus, Micrococcus luteus, Pseudomonas aeruginosa, Escherichia coli (E. coli), Aspergillus fumigatus and Aspergillus niger [18]. Also Rosemary et al obtained results using a complex made of ciprofloxacin and gold nanoshells with high antibacterial activity against E. coli [19]. In a study conducted by Gu et al stable water soluble vancomycin covered with AuNPs as polyvalent inhibitors was prepared and demonstrated their effectiveness towards various enteropathogenic strains, such as vancomycin-resistant strains (VRE) and gram-negative bacteria [20].
It has been reported also that AuNPs possess unique chemical and physical properties for transporting and unloading the pharmaceuticals. The first advantage of AuNPs as a drug carrier is that the gold core is essentially inert and non-toxic [21], also the choice of AuNPs is favored by the ease of synthesis and its readiness in functionalization, generally through thiol linkages. Above all, its photo-physical properties could trigger drug release at remote place [22]. One of the processes used in AuNPs synthesizes is reduction process. Reduction process can take place either in chemical or biological synthesis method in which a stabilizer (capping/stabilizing agent), metal precursor, and reducing agents are the basic component needed; the major stabilizing agents are polymers, citrate [23], phosphine [24], and alkanethiol [25]. In chemical method, the metal precursor is majorly the metallic salt of the nanoparticles to be produced; for AuNPs, the metal precursor is chloroauric acid (HAuCl4) and the reducing agents majorly used are sodium citrate, sodium borohydride [26], glucose [27], and ethanol [28]. This was shown in chemical synthesis of AuNPs by Manson et al using sodium citrate, sodium borohydride and also hydroquinone to reduce chloroauric acid (HAuCl4) [12]. Biological methods of nanoparticle synthesis also involved the usage of same type of metal precursor but different reducing agents. Micro-organisms, enzymes, and plant extracts as reducing agents are most paramount in biological method [29]. Using plants for nanoparticle synthesis can be advantageous over other biological processes because it eliminates process of maintaining cell cultures and is suitable for large scale nanoparticle synthesis [30]. It has been reported that AuNPs of size 5–100 nm was synthesized by using buds of Syzygium aromaticum and it was found to be crystalline in nature. The AuNPs with average particle size of 300 nm was synthesized using banana peel extract and confirmed by different techniques [31]. Mentha piperita extract was used to synthesis of spherical shaped gold nanoparticles with size around 150 nm and it showed antimicrobial activities against S. aureus and E. coli [32]. The extract of Madhuca longifolia was used to reduce the AuNPs [33] and biological procedure using biomass of Suaeda monoicaleaves was reported with particle size ranged from 3.89 to 25.83 nm with average particle size of 12.96 nm. The leaves extract of Stevia rebaudiana for the reduction of gold ions to nanoparticles was studied and spherical shaped nanoparticles with size range from 5 to 20 nm were synthesized [34]. The biological AuNPs were synthesized by using the leaf extract of Coleus amboinicus with size ranged from 4.6 to 55.1 nm. The AuNPs with a particle size ranging from 5 to 15 nm were synthesized using Zingiber officinale extract which acts both as reducing and stabilizing agent [35]. The extract of Pistacia integerrima was also used for synthesizing AuNPs in range of 20–200 nm [36]. A case study of plant used in this work is Piper guineense (plate 1). Piper guineense is an African spice plant and is commonly called Ashanti pepper. It is also referred to as climbing black pepper, Benin pepper, or bush pepper. It belongs to the plant family called Piperaceae [37]. Piper guineense is added to food for pregnant and nursing mothers as a medicinal spice and among the post-partum women; it is claimed that it assists in the contraction of the uterus [38]. It is generally known to possess antimicrobial and antioxidant properties [39]. The medicinal properties of Piper guineense exert bacteriostatic and bacteriocidal effects on some bacteria. The leaves are considered appetitive, carminative and eupeptic. They are also used for the treatment of cough, bronchitis, intestinal disease and rheumatism [40]. It has been reported that the leaf extracts of Piper guineense has antimicrobial effect on these test organisms: S. aureus, E. coli, Candida albican and Aspergillus flavus [41]. The phytochemical composition of parts of this plant has been determined; the seeds have been reported to contain high amounts of cardiac glycosides, tannins, flavonoids and terpenes [40]. Also reported are those of the leaves which also contain high amounts of tannins, flavonoids, and alkaloids [41]. Also required for AuNPs synthesis is a polymer that will be used as capping agent that will aid the stability of the nanoparticle.
Plate 1. Leaf and fruit of Piper guineense.
Download figure:
Standard image High-resolution imageA polymer (capping agent) such as polyethylene glycol (PEG) is normally used to prevent degradation before delivery though it is a water soluble polymer; it has ability of compatibility with the immune system of the human body with non-immunogenicity, non-antigenicity, and protein rejection properties [42, 43]. PEG is preferred in the biomedical field and is referred to as oligomers and polymers with a molecular mass below 20 000 g mol−1 [44]. PEG through the process of PEGylation is introduced for functionalization of nanoparticles and it is suitable for biological applications because of its solubility in water and has low intrinsic toxicity [44]. The main advantages of PEG-drug conjugates are reduced protein immunogenicity, increased residence time in the body, reduced enzymatic degradation. All these features ensure that the drug reaches the site of action and prevents clearance from the body because it is not recognized as the foreign body [45]. The high hydrophilic nature of PEG enhances the solubility of hydrophobic drugs or carriers when conjugated with them. It enhances the physical and chemical stability of drugs and prevents aggregation of the drugs in vivo, as well as during storage, as a result of the steric hindrance and/or masking of charges provided through formation of a conformational cloud [46]. To test the performance of AuNPs produced, lincomycin was loaded on the functionalized AuNPs and the influence of temperature in the release efficiency was investigated.
Lincomycin as a drug, fights bacteria and it is used to treat severe bacterial infections in replacement of penicillin antibiotics [47]. Lincomycin is a lincosamide antibiotic which blocks protein synthesis in sensitive bacterial strains by inhibiting the peptide transfer reaction on the 50S ribosomal subunit [48]. Therefore, the bioreduction capability of Piper guineense aqueous leaf extract on tetra gold chloride into gold nanoparticles and its ability as drug carrier with covalent functionalization with polyethylene glycol and its encapsulation with standard drug (lincomycin), to give a product (nanodrug) was investigated in this work.
2. Materials and methods
2.1. Materials
All chemicals used in these work are of analytical grade with percentage purity in the range of 99.8%–99.9%. Hydrogen tetra auric acid (HAuCl4) and polyethylene glycol (MW 3000) were obtained from Sigma Aldrich Company, USA. The distilled and deionized water used were gotten from Centre for Genetic Engineering and Biotechnology, Federal University of Technology Minna, Nigeria. The leaves of Piper guineense (plate 1) was obtained from Enugu State, Nigeria. The micro-organisms used were obtained from the Department of Microbiology, Federal University of Technology Minna, Nigeria. These organisms are S. aureus, E. coli and S. pyogenes.
2.2. Methods
2.2.1. Synthesis of gold nanoparticles.
Fresh leaves of Piper guineense were washed with distilled water, and then air dried for 15 d at room temperature to prevent the destruction of thermo labile constituent of the plant by direct sun rays. The leaves were then milled into coarse powder. After which 5 g of the plant sample (Piper guineense) was weighed and added to 100 ml of sterile distilled water in erlenmeyer flask and boiled for 5 min and filtered. The qualitative phytochemical analysis was carried out on the plant extract according to the methods of [49, 50] to confirm the presence of alkaloids, saponin, flavonoids, cardiac glycosides, tannins, steroids, phlobatannin, terpenoids and anthraquinones. About 0.5 ml of the filtrate was pipetted into a test tube and 9.5 ml of 1 mM HAuCl4 added for the reduction of Au3+ ions to Au0 (equation (1)) according to the method described by Sing [51]. Colour change was observed and UV–Vis spectrophotometer was used to determine the wavelength.

2.2.2. Characterization of biosynthesized AuNPs.
The biosynthesized AuNPS were characterized by malvern nanozetasizer (nano-S model) to determine its particle size, transmission electron microscope (TEM) performed on a JEOL model 1200EX instrument at University of Western Cape, South Africa. It was operated at an accelerating voltage at 80 kV to determine its morphology; shape, size and purity. The elemental composition was analyzed on energy dispersive x-ray spectroscopy (EDS) S-3400N, and Fourier transform infrared spectroscopy (FTIR) to assess the functional groups functionalized AuNPs. The UV-spectroscopy measurement of the HAuCl4—plant extract solution was carried out using a UV-1800 Shimadzu spectrophotometer. The various peaks present in the mixture was measured over a range of 300–800 nm and the absorbance of the mixture was measured at various temperature (40 °C, 60 °C, 80 °C and 100 °C) and time within the range of 0–60 min.
2.2.3. Functionalization of biosynthesized AuNPs.
The functionalization of the AuNPs was carried out using a standard drug (lincomycin) and polyethylene glycol. Different formulations were formed from three individual components to form three different composites. The first formulation (composite of polyethylene glycol, gold nanoparticles and the drug (PND)) was prepared by the addition of 0.5 ml of biosynthesized AuNPs with 5 g polyethylene glycol and 0.58 g of the lincomycin with proper stirring for 1 h using magnetic stirrer. Second formulation (composite of polyethylene glycol and gold nanoparticles (PN)) was obtained using 0.5 ml of biosynthesized AuNPs added to 5 g polyethylene glycol and stirred for 1 h. The third formulation (composite of polyethylene glycol and the drug (PD)) was gotten by 1 ml sterile deionized water mixed with 0.58 g of standard drug and 5 g of polyethylene glycol with proper stirring of 1 h. All the formulations were made into tablet and left for 24 h in an incubator at 37 °C to enable wet digestion of the mixture, and then properly air dried (equation (2)).
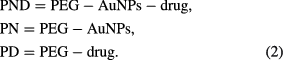
2.2.4. Drug release.
The drug release was carried out in a sterile environment. Test tubes containing 3 ml sterile deionized water were set up for each formulation. The height and diameter of each tablet were measured and placed in a 3 ml sterile deionized water for 3 min, then removed and the height and diameter were then measured, the process was repeated at various time intervals until the whole tablets dissolve, with the standard drug concentration and absorbance of the various formulations were measured with the UV–Vis spectrophotometer.
2.2.5. Antibacterial assay.
A known weight of the nutrient agar was dissolved in 300 ml sterile distilled water and 10 cm3 of nutrient broth was dispensed in three test tubes for each organism. This was then autoclaved at 121 °C for 15 min. The deionized water used in drug release was also sterilized at same condition. After sterilization, they were allowed to cool to about 45 °C and the nutrient agar was then dispensed into petri dishes, which were left to solidify. Holes were then made on the agar containing petri dishes using a sterile cork borer with 10 mm diameter. The organisms were then inoculated into the nutrient agar plate, and then 2 ml of each of the dissolved formulated drugs (PND, PD, and PN) were dispensed into the holes made. The petri dishes were left to stand for 2 h and then placed in the culture box for 24 h. After incubation the diameter of inhibition zone (mm) were measured.
2.2.6. Statistical analysis.
The data were statistically analyzed using one-way analysis of variance (ANOVA) and Duncan multiple range test. Values are represented as mean ± standard error of mean. Data from the antibacterial activities were compared with their respective controls and differences at p < 0.05 were considered significant.
3. Results and discussion
3.1. Qualitative phytochemical constituent of Piper guineense
The qualitative phytochemical composition of the aqueous leaf extract of Piper guineense is presented in table 1, which indicates the presence of tannins, flavonoids, anthraquinones, phlobatannins while saponins and alkaloids were absent.
Table 1. Phytochemical composition of aqueous extract of Piper guineense leaf.
Phytochemicals | Inference |
---|---|
Tannins | + |
Flavonoids | + |
Anthraquinones | + |
Saponins | − |
Phlobatannins | + |
Alkaloids | − |
Terpenoids | + |
Cardiac glycosides | + |
Keys: (+) present, (−) absent.
The phytochemical screening of the aqueous extract of Piper guineense showed the presence of tannins and flavonoids (table 1) which are examples of polyphenolic compounds that are of great importance to green synthesis of nanoparticles due to their reductive and antioxidant abilities. Tannins has been reported as reducing and stabilizing agents to synthesize silver nanoparticles on a timescale of seconds through a simple, green, room temperature protocol, and the suitability of this protocol for energy-efficient, continuous flow synthesis has been demonstrated using a simple co-axial flow reactor [52]. The flavonoids presents also were found to be responsible for reduction process to produce AuNPs [52]. The absence of alkaloids and saponins shows that the Piper guineense does not contain compounds with basic nitrogen atoms and foaming characteristic which basically are important for nanoparticle formation while the presence of tannins and flavonoids aids in the reduction of the metal precursor to synthesize AuNPs.
3.2. Biosynthesis of gold nanoparticles with aqueous leaf extract of Piper guineense
The gold chloride solution was yellow in colour (a) before the reduction reaction; the aqueous extract of Piper guineese is deep orange (b) in colour while the ruby colouration (c) formed after the reduction reaction of the aqueous extract of Piper guineense on the gold chloride solution to form biosynthesized gold nanoparticles as shown in plate 2.
Plate 2. (a) Gold chloride solution; (b) Piper guineense and (c) gold nanoparticles.
Download figure:
Standard image High-resolution imageA relationship was noticed with temperature, reaction rate and color intensity. It was noticed that as temperature increases, the rate of reaction also increases, with a change in colour to ruby red (plate 2(c)). This corresponds to the previous research carried out by Jae et al [30] where it was reported that at 95 °C, only 3 min was needed for 95% reduction of gold chloride to gold nanoparticles. The sample produced was characterized with UV–Vis spectroscopy to detect the surface plasmon resonance (SPR) through the absorption peak of the biosynthesized AuNPs, which was observed at 552 nm as shown in figure 1. The presence of phenolic groups in Piper guineense has brought about the reduction of the tetra gold chloride to gold nanoparticles, with a change in color (plate 2(c)). Unreduced tetra gold chloride, absorbs at 300 nm, and gold nanoparticles absorbs within the range of 500–600 nm. The UV–Vis spectrophotometer confirmed the reduction, as the peak of the biosynthesized gold nanoparticles from Piper guineense was observed to be 552 nm (figure 1), Umesh et al [29] reported the peak for the synthesis of gold nanoparticles with Allium cepa to be 540 nm.
Figure 1. Absorption spectra of (a) tetra gold chloride and (b) biosynthesized gold nanoparticles.
Download figure:
Standard image High-resolution image3.3. Particle size dynamic light scattering of biosynthesized AuNPs
The size distribution (hydrodynamic radius) of the synthesized AuNPs were measured using nano-zetasizer dynamic light scattering (DLS). Results obtained as presented in figure 2 indicate that the average particle size in diameter of the biosynthesized AuNPs are 197.2, 220.8, 187.2 and 242.9 nm at various temperatures 40 °C, 60 °C, 80 °C and 100 °C, respectively.
Figure 2. Effect of temperature on particle size of the biosynthesized AuNPs.
Download figure:
Standard image High-resolution imageIn this work, there was no correlation between the particle size and temperature; this contradicts the work of Jae et al, who reported that increase in temperature, brought about a decrease in size [30]. The particle size is a contributory factor to the drug release and transportation to the target area, therefore the functionalized AuNPs with its particle size ranging from 187.2 nm–242.9 nm has high capacity of achieving high ligand density on the surface as a carrier for delivering purposes.
3.4. Transmission electron microscopy of the functionalized AuNPs
The transmission electron microscopy (TEM) monograph of functionalized nanoparticle is shown in figure 3. Gold nanoparticles exhibits various size ranges as well as different shapes such as spherical, octahedral, sub-octahedral, decahedral, multiple twined, icosahedral multiple twined, irregular shape, tetrahedral, nanotriangles, nanoprisms, hexagonal platelets and nanorods [2]. The image revealed the shape to be spherical and triangular which are majorly used for drug delivery, photo-thermal therapy and refractive-index sensing.
Figure 3. TEM images of the functionalized AuNPs at different scale bar.
Download figure:
Standard image High-resolution imageAmong all these shapes, the triangular shaped nanoparticles show attractive optical properties as compared to the spherical shaped nanoparticles [2]. The TEM images in figure 3 also show that the standard drug (lincomycin) was properly encapsulated into the functionalized spherical and triangular AuNPs for the purpose of target delivery. The functionalized nanodrug was investigated using EDS and confirmed the presence of gold nanoparticles as shown in figure 4. The vertical axis shows the number of x-ray counts and the horizontal axis shows energy in keV. The maximum optical adsorption peak was observed at approximately 2.30 keV and addition signals for carbon at 0.20, oxygen 0.50 and copper 1.0.
Figure 4. EDS spectrum of functionalized AuNPs.
Download figure:
Standard image High-resolution imageThe peaks situated at bonding energies of approximately 1.2, 8.3 and 9 keV belong to copper while the first peak belong to carbon. The presence of copper and carbon as revealed by the EDS corresponds to the TEM holding grids, though the elemental carbon could also be from the carboxyl group in the precursor (Piper guineense extract). Also, it can be deduced from the TEM/EDS results that the synthesized gold nanoparticles have high purity.
3.5. FTIR of functionalized gold nanoparticle
The FTIR spectra (figure 5) of gold nanoparticles synthesized under optimized conditions indicated the presence of the functional groups and the biomolecules in the gold nanoparticles with strong signals between 3434.37–3417.01 cm−1 and weak signals at 1643.41 cm−1.
Figure 5. FTIR spectrum of biosynthesized gold nanoparticles.
Download figure:
Standard image High-resolution imageThe FTIR showed strong bands between 3434.37–3417.01 cm−1 a characteristic band of hydroxyl functional groups in alcohols and phenolic compounds. This is additional evidence that the phenolic phytochemicals are acting as reducing agents. Jae et al [30] also reported to have strong bands at 3332 cm−1, linking to the phenolic phytochemicals present in Magnolia kobu. The weak signals at 1643.41 cm−1 corresponds to alkene groups.
3.6. Drug release
The release capability of nano-formulated drug of PND, PD and PN at the temperatures 40 °C and 60 °C are represented in figures 6 and 7, with PND having the highest release capacity with peak at 9 min (23.4 mg ml−1 for 40 °C) and (29.5 mg ml−1 for 60 °C).
Figure 6. Release efficiency of formulated drug at 40 °C.
Download figure:
Standard image High-resolution imageFigure 7. Release efficiency of formulated drug at 60 °C.
Download figure:
Standard image High-resolution imageThe entire functionalized drug (PND, PN and PD) showed a progressive increase in the concentration of their drug release, with maximum peak at the 9th min. The formulations containing all three components (PND) had the highest standard drug release, as compared to other formulations. This implies that the increase in the rate of the standard drug release is the attribute of both the PEG and the AuNPs effect. But more of the induction is from the nanoparticle rather than the polymer.
3.7. Antibacterial activities
The formulated drug was assessed on two different bacteria, two gram positive (S. aureus and S. pyogenes). The zone of inhibitions of the two formulations at 40 °C (PND and PD) with respect to the standard drug (lincomycin) on S. aureus (figure 8) and S. pyogenes (figure 9).
Figure 8. Antimicrobial potential of formulated nanodrug (40 °C) for S. aureus.
Download figure:
Standard image High-resolution imageFigure 9. Antimicrobial potential of formulated nanodrug (40 °C) for S. pyogenes.
Download figure:
Standard image High-resolution imageHowever, the zone of inhibitions of two formulations at 60 °C (PND and PD) with respect to the standard drug (Lincomycin) on S. aureus and S. pyogenes were shown in figures 10 and 11, respectively.
Figure 10. Antimicrobial result of functionalized gold nanodrug (60 °C) for S. aureus.
Download figure:
Standard image High-resolution imageFigure 11. Antimicrobial result of functionalized gold nanodrug (60 °C) for S. pyogenes.
Download figure:
Standard image High-resolution imageThe standard drug denoted as SD (Lincomycin, 100 mg ml−1) zone of inhibition of growth was 29 mm for S. aureus (figures 8 and 10) and 27 mm for S. pyogenes (figures 9 and 11) but it did not exceed 3 min while other type of drugs lasted longer in their inhibition activities. The PND formulation had highest inhibitions of 18 mm (at 40 °C) and 16 mm (at 60 °C) for S. aureus, and 16 mm for S. pyogenes (both at 40 °C and 60 °C). The bacteria growth inhibition continued and lasted for 15 min with respect to PND, while that of PD only lasted for 9 min with lesser growth inhibition compared to PND. Temperature was also a factor that affects inhibition because from this work, it was observed that lower temperature (40 °C), there was greater growth inhibition of bacteria (18 mm) compared to at temperature of 60 °C with growth inhibition of bacteria to be 16 mm for S. aureus. The results in this work imply that the presence of the gold nanoparticle increased the efficiency of the standard drug (Lincomycin), and so, even at a lower drug concentration compared to the standard, a growth inhibition occur thereby increasing its effectiveness.
4. Conclusion
This work reveals that Piper guineense has the ability to serve as a reducing agent for the green synthesis of gold nanoparticle and that in vitro dissolution methods developed to evaluate the potential of the in vivo performance of the formulated nanodrug demonstrate the unique ability and capacity of polymer-nanoparticle encapsulated drug; as a drug delivering agent that enhances the property of the drug towards a better performance. Therefore, gold nanoparticle has a great potential in drug delivery and also aid in the efficiency of the drug.
Acknowledgments
We greatly acknowledge the Tertiary Educational Trust Fund of Nigerian for TETFUND-Institutional, Based Research Grant (TETFUND/FUTMINNA)/2015/31). The Centre for Genetic Engineering and Biotechnology, Federal University of Technology, Minna is also thanked for enabling direct access to the center facilities..